I. Epigenetics
is the study of changes in organisms caused by modification of gene expression rather than alteration of the genetic code itself, and is part of biology that explains what modifications may occur in our genome and gene expression due to environmental and genetic factors. One epigenetic expression is methylation which is the change or modification in dna expression. Epigenetic factors, such as better diet, eating fresh, exercise, sleep, environment , may also revert DNA modifications.
The main part of epigenetics is chromatin regulation that has been proved to be essential to genomic processes including DNA damage. Chromatin structure can be modified by enzymes and factors.
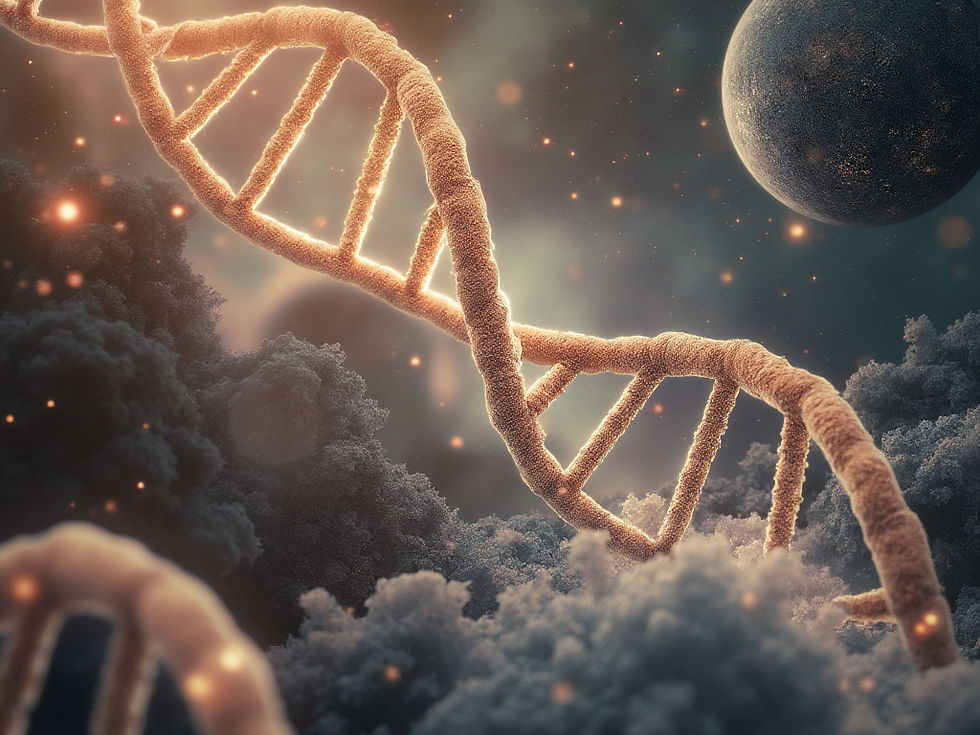
Our genome is made of four letters - A, T, C, G. But those four letters are used over and over again in billions of long sequences. Sometimes it gets only one of those letter to be modified that can lead to a devastating disease.
There are three molecular changes that lead to epigenetic changes Histon modification, chromatin methylation, non-coding RNA. Those lead to either silencing of genes or expression of such.
II. Chromatin structure
The main composition of chromatin is DNA and strictly packed histones.
Genomic DNA is folded in small nucleus through proteins called histones, where the DNA is wrapped around those histones. The nucleosome contains histone proteins and 146 base pairs of DNA warped twice around the histone. Core histone proteins are H2A, H2B, Histone3, Histone4. Histones fold and form different forms. Histone tails are unstructured. They are quite long and play a role in further folding. They are hard positively charged and can lead to other particles.

III. Switching genes
on or off plays a crucial role in epigenetics. Here's how this switching mechanism works within the realm of epigenetics:
1. DNA Methylation: This is the addition of a methyl group to the DNA, typically at cytosine bases that are followed by a guanine (CpG sites). Methylation usually acts to repress gene transcription, meaning it turns genes off. When a gene is methylated, the cellular machinery has a harder time reading the gene, thus reducing or silencing its expression.
2. Histone Modification: DNA is wrapped around proteins called histones. Chemical modifications to these histones, like acetylation, methylation, phosphorylation, and ubiquitination, can change how tightly DNA is wound.
Acetylation generally promotes gene expression by loosening the DNA-histone interaction, making DNA more accessible for transcription.
Histone acetylation contributes to changes in chromatin structer and it also creates binding sites for co-factors, f.e protein, means further acetylation, called hyper-acetylation. Nucleosome remodelling is also accelerated, that can disrupt the nucleosome and make it unstable.
Methylation of histones can either activate or repress genes depending on which amino acids in the histones are methylated and how many methyl groups are added.
Histone methylation- HMT(histone methyl transferase) is linked to lysine demethylase whicleads to lysine being either demethylated or mono, di or try-methylated. Lysine residues can be methylated multiple times. Different states have different functional consequences. Only deacetylated lysins can be methylated. Meaning that acetylation and methylation is not possible together. Histone methylation is again associated with transcription activation or repression. How does it works? Lysin methylation creates binding sites for co-activators,some chromatin remodelling enzymes.
3. Chromatin Remodeling: Chromatin is the complex of DNA and proteins that condenses to form chromosomes. Remodeling complexes can change the structure of chromatin to expose or hide genes, thereby affecting gene expression without changing the DNA sequence.
4. Non-coding RNAs: These include microRNAs (miRNAs) and long non-coding RNAs (lncRNAs). They can influence gene expression post-transcriptionally. For example, miRNAs can bind to messenger RNA (mRNA) and prevent translation or lead to degradation of the mRNA, effectively switching off the gene's protein product.
5. Gene Silencing: Through RNA interference (RNAi), small RNA molecules can lead to the degradation of specific mRNA molecules or block their translation, thus silencing gene expression.
6. Imprinting: Certain genes are expressed in a parent-of-origin-specific manner, which means that only the maternal or paternal allele of the gene is expressed while the other is silenced through epigenetic marks.
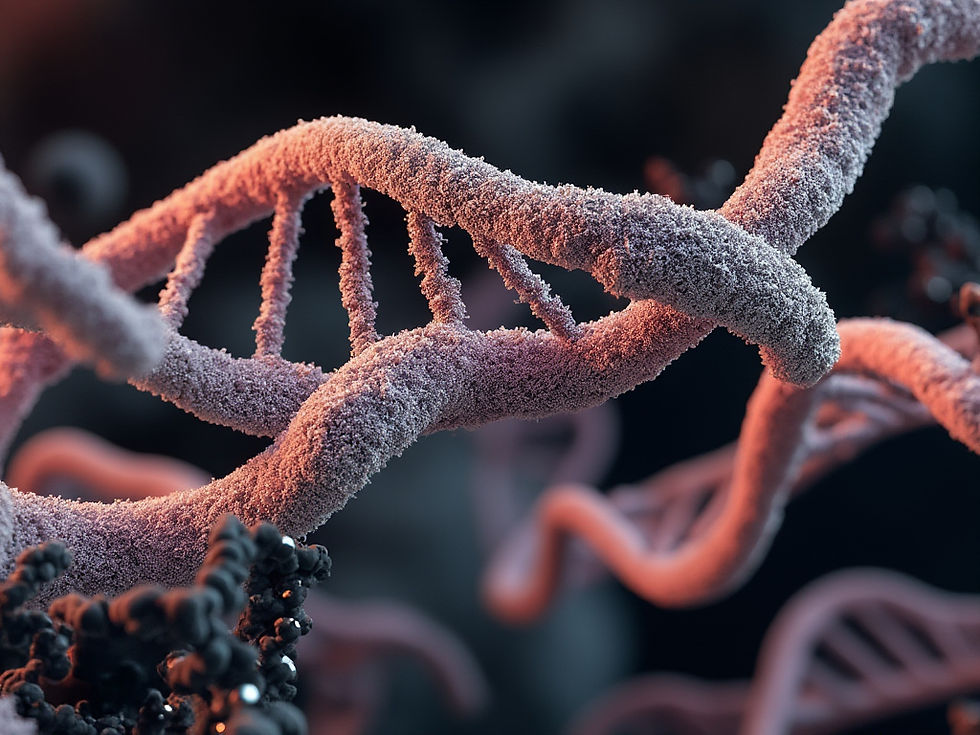
IV. Behavioral epigenetics
is a field of study that explores how epigenetic changes — modifications in gene expression that do not involve changes to the underlying DNA sequence — influence behavior, and how behaviour, in turn, can influence epigenetic states. Here are some key points about behavioral epigenetics:
1. Mechanisms: This field examines how environmental factors, social experiences, stress, diet, toxins, and other external influences can lead to epigenetic modifications like DNA methylation, histone modification, and changes in non-coding RNA, which then affect gene expression.
2. Impact on Behavior: These epigenetic changes can affect neurobiology, influencing behaviors such as:
Learning and Memory: Epigenetic mechanisms can alter how memories are formed, stored, and retrieved. For instance, histone acetylation can facilitate memory formation by making genes more accessible for transcription.
Stress Response: Exposure to stress, especially early in life, can lead to epigenetic changes that affect the stress response system (e.g., the hypothalamic-pituitary-adrenal axis), potentially leading to altered stress reactivity throughout life.
Mental Health: There's a growing body of evidence suggesting that epigenetic modifications play roles in mental health disorders like depression, anxiety, schizophrenia, and addiction by altering gene expression in brain regions involved in mood regulation and cognitive function.
Social Behavior: Social interactions, including maternal care, can induce epigenetic changes that influence social behavior, attachment, and even aggression.
3. Transgenerational Epigenetics: Some research indicates that epigenetic marks can be inherited, suggesting that the behaviors or environmental exposures of parents (and potentially grandparents) could affect the behavioral tendencies of offspring through epigenetic mechanisms, although this area is still controversial and under active investigation.
4. Reversibility: One of the exciting aspects of epigenetics, in contrast to genetic mutations, is that epigenetic changes are potentially reversible. This opens up therapeutic possibilities where interventions, whether behavioral or pharmacological, could alter or reverse detrimental epigenetic marks.
5. Examples in Research:
Rat Maternal Care Studies: Research has shown that the amount of licking and grooming a rat pup receives from its mother can influence the pup's epigenetic state, particularly in genes related to stress response, which in turn affects its behavior as an adult.
Human Studies: Epigenetic changes have been observed in individuals who experienced trauma or severe stress, like survivors of child abuse or war, potentially linking these experiences to long-term behavioral outcomes.
Behavioral epigenetics provides a biological framework for understanding how life experiences can become biologically embedded, influencing an individual's behavior, health, and potentially even the health and behavior of their descendants. This field is at the intersection of genetics, psychology, neuroscience, and environmental science, offering insights into how nurture shapes nature.
V. More Than Just Our Genes
The age-old debate about nature versus nurture has long captivated scholars, scientists, and philosophers. For much of the 20th century, genetic determinism held sway, suggesting that our genes alone dictate our physical traits, behaviors, and susceptibilities to diseases. However, the advent of epigenetics has transformed this narrative, illuminating a profound truth: we are indeed more than just our genes.
Epigenetics, literally meaning "above" or "on top of" genetics, explores mechanisms that regulate gene expression without altering the DNA sequence itself. This field reveals that our genes are like the keys on a piano, but epigenetics determines which keys are played, how hard they are struck, and for how long. Here's how epigenetics underscores our existence beyond mere genetics:
Environment's Echo: Our immediate environment, from the air we breathe to the stress we endure, can imprint on our genetic material. For instance, pollutants or stress can lead to epigenetic marks like DNA methylation, potentially silencing genes that should be active or vice versa. This interaction shows that our surroundings have a direct, albeit invisible, conversation with our DNA.
Nutritional epigenetics has shown that what we eat can influence our gene expression. Certain nutrients can methylate DNA or modify histones, influencing everything from metabolism to mental health. The old adage "you are what you eat" gains a literal dimension, suggesting that dietary choices can shape not just our physique but our genetic activity.
Behavioral epigenetics examines how our behaviors and experiences, like exercise or trauma, can cause epigenetic changes. For example, regular physical activity has been shown to alter the epigenome in muscle cells, improving metabolism and potentially reducing the risk of various diseases. This implies our lifestyle choices are not just immediate choices but are scripting our genetic expression.
Understanding that we are more than our genes has significant implications for health and disease:
Preventive Measures: If epigenetic changes are reversible, then lifestyle interventions could potentially undo harmful epigenetic marks, offering new avenues for preventing diseases before they manifest.
Therapeutic Frontiers: Drugs that target epigenetic mechanisms are already in use for certain cancers, showing that altering gene expression can be as effective as altering the genes themselves.
The revelation that we are more than our genes invites us into a world where biology is not destiny but potential. Epigenetics offers a narrative where our actions, our choices, and our environments are continuously interacting with our genetic legacy, offering us a canvas rather than a fixed script. It empowers us with the knowledge that through our behaviors, diets, and by shaping our environments, we have a say in how our genes express themselves. This dynamic interplay between genetics and epigenetics redefines individuality, health, and heritage, encouraging a holistic view of life where we are both the artist and the art, actively participating in the unfolding story of our genetic expression.
Controlling gene expression epigenetically can be achieved though control over our environment, exercise and diet, stress management and exposure to clean air as well as increase in attention over edpigenerics drugs such as HDAC Inhibitors and DNMT Inhibator. HDAC Inhibators: Drugs like vorinostat (SAHA) and romidepsin are used to inhibit histone deacetylases, leading to increased acetylation and thus more active gene expression. These are used in cancer treatments to reactivate silenced tumor suppressor genes. DNMT Inhibitors: Azacitidine and decitabine inhibit DNA methyltransferases, reducing DNA methylation. They're used in treating myelodysplastic syndromes and leukemia by reactivating genes that have been inappropriately silenced.
VI. Introduction to Anti Aging
Epigenetic can be run backwards so that it drives modification of the aging process. Epigenetics may be one of the factors that will help scientists of anti-aging and be an additional to the cocktails of hosrmocs and stemm cells regeneration and longevity. Chemical-based reprogramming is promising for safer epigenetic rejuvenation approaches. Certainly, interventions that target epigenetic factors have shown success in reducing the effects of aging or extending life in various animal studies. Techniques involving small molecules and reprogramming approaches that promote epigenetic renewal have been created to improve or reverse conditions linked to aging. Furthermore, engaging in lifestyle changes like limiting calorie intake, regular exercise, and regulating sleep cycles has proven effective in slowing down the aging process.
NAD+ is essential for aging due to its role in DNA repair and epigenetic regulation. Its levels can be boosted with precursors like NMN, NR, and NAM, which help prevent NAD+ decline and combat aging and related diseases. These supplements have shown to extend life, enhance mitochondrial function, delay aging effects in various animal models, and improve conditions like cognitive decline in Alzheimer's models. However, more clinical research is needed to confirm these benefits in humans.
Reprogramming (Source: Nature) somatic cells with Yamanaka factors (Oct3/4, Sox2, Klf4, and c-Myc; OSKM) reverses cell fate and finally generates induced pluripotent stem cells (iPSCs), which possess the characteristics of ESCs.288,289 A classic strategy for combating aging comes from the generation of iPSCs. The durable expression of OSKM leads to widespread chromatin remodeling,290 and interestingly, some aged somatic cells can be reprogrammed to exhibit a youthful state. Ectopic expression of OSK without c-Myc restores the young patterns of DNA methylation and transcriptomes in mouse retinal ganglion cells, which can ameliorate vision problems in glaucomatous in aged mice. The DNA demethylation induced by OSK expression is confirmed to be necessary for the rejuvenation process of retinal ganglion cells.291. Transfecting aged human fibroblasts, chondrocytes, and endothelial cells with mRNAs expressing OSKMLN (OSKM, LIN28 and NANOG) rejuvenates host cells and significantly reverses the epigenetic clock.295 More recently, a 13-day OSKM reprogramming using the Tet-on expression system significantly reduced the epigenetic age of human fibroblasts without fully changing them into iPSCs, indicating a boundary between the rejuvenation and the pluripotency programs.296 Furthermore, short-term expression of OSKM in vivo significantly expands the lifespan of progeria mice and restores the levels of H3K9me3 and H4K20me3.292,297 In addition, a 2.5-week transient reprogramming in early life (2-month-old mice) is sufficient to extend the lifespan of transgenic progeria mice by 15% and rejuvenates the DNA methylation patterns in skin cells.298 The aging-associated epigenetic and transcriptional changes can also be alleviated by transient reprogramming in naturally aged mice.299 Overall, both long-term and transient reprogramming can achieve the rejuvenation of aged cells, while transient reprogramming also provides a novel method to alleviate aging in vivo in an organism.
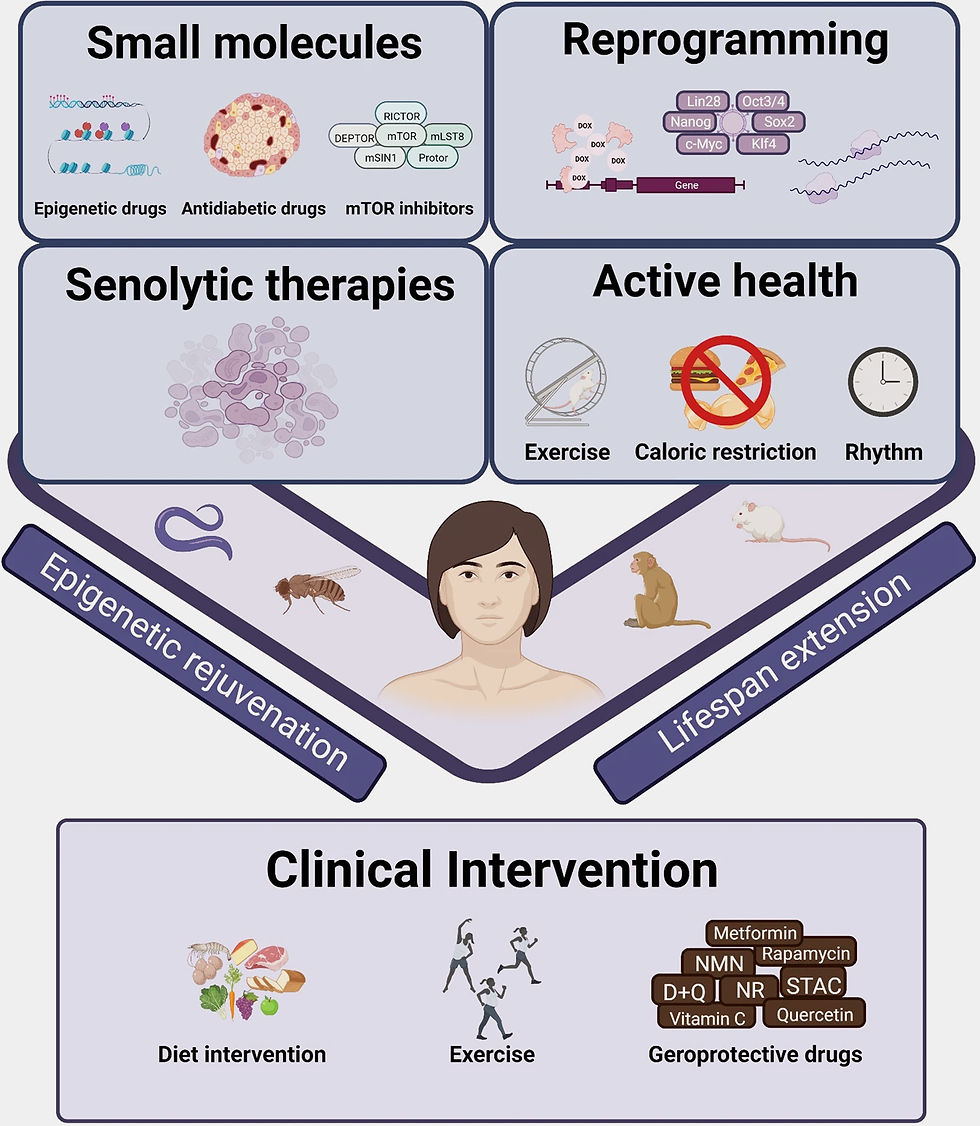
Exercise, caloric restriction, cardiac rhythm, day-night clock have shown an enormous importance when it come to taking visible control over prolonged life.
Studies in C. elegans, Drosophila, and mammals have unraveled the aging-related epigenetic changes in DNA, RNA, and histone modifications and alterations in the more advanced chromatin structure states. Correspondingly, these epigenetic changes have been identified as biomarkers or intervention targets of aging, such as the global decrease in genomic DNA methylation, the global loss of canonical histones, chromatin landscape remodeling caused by heterochromatin loss, and nuclear membrane protein changes in human and mouse tissues during aging. However, the same chromatin modifications (e.g., H3K14ac and H3K27me3) may play opposite roles in regulating aging and longevity across species and even across tissues within the same species, indicating that epigenetic changes need to be interpreted with their context. It is noteworthy that emerging technologies such as single-cell omics sequencing provide a higher resolution for dissecting epigenetic characteristics during aging, and provide new avenues for investigating the heterogeneity of aged cells. In addition, the spatiotemporal transcriptomic atlas across multiple mammalian tissues can provide more information on aging-related interactions between cells or tissues, which may facilitate the design of better and more precise therapeutics for aging and aging-related diseases.
Here's an overview of the main factors contributing scientifically to anti-aging (Supported by Grok| xAI)
Genetic and Epigenetic Factors: Genetic Interventions: Research into genes like Klotho, SIRT1, and others associated with longevity could lead to therapies that mimic the effects of these genes, potentially extending life or improving health during aging.
Epigenetic Modifications:Epigenetics involves changes in gene expression without altering the DNA sequence. Interventions here could reverse some aging effects by resetting epigenetic markers.
Mitochondrial Health:
Mitochondrial Function: Improving mitochondrial health through various compounds or lifestyle changes can reduce oxidative stress, a key factor in aging.
Cellular Senescence: Senolytics: These are drugs designed to clear senescent cells, which accumulate with age and contribute to aging and age-related diseases.
Nutrient Sensing and Diet:
Dietary Interventions: Caloric restriction or diets that mimic its effects (like intermittent fasting) influence aging through pathways like mTOR, which when inhibited, can lead to increased longevity in various organisms.
Inflammation Reduction: Anti-inflammatory Approaches: Diets or drugs that reduce systemic inflammation are considered anti-aging because chronic inflammation is linked to many age-related diseases.
Telomere Maintenance:
Telomerase Activation:Though controversial due to potential cancer risks, maintaining telomere length could theoretically slow aging.
Proteostasis: Protein Homeostasis: Ensuring that proteins within cells are properly folded and functional, and removing those that aren't, can prevent diseases like Alzheimer's, which are linked to aging.
Microbiome:
Gut Health: A balanced gut microbiome influences aging by affecting nutrient absorption, inflammation, and even mental health.
Physical Activity: Exercise: Regular physical activity, especially aerobic and resistance training, helps maintain muscle mass, cardiovascular health, and can influence epigenetic markers positively.
Stem Cell Therapy
Regenerative Medicine:Stem cells can differentiate into various cell types, offering potential treatments for aging tissues and organs. Therapies might involve direct transplantation or techniques to rejuvenate one's own stem cells. Recent discussions on platforms like X highlight both the excitement around stem cell therapies for conditions like diabetes and the controversies regarding their effectiveness and ethical considerations.
Exosomes and Stem Cell Secretome: Instead of using stem cells directly, using the factors they secrete (exosomes) could provide anti-aging benefits without the risks associated with cell transplantation.
Each of these areas represents a frontier in anti-aging research, with stem cell therapy particularly capturing public and scientific interest for its regenerative potential. The need for more therapy options and more research to validate efficacy and long term future prospective. It sounds insane yet lovely knowing reversion and science.
Image sources: 1/Grok xAI 2/Nature(epigenetic rejuvenation) 3/BC Campus(Chromatin structure) 4/EdMine Gallery
Blog essay post source: 1/Epigenetics:More than just our Genes(A course by University of Cambridge), 2/Nature 3/Grok xAI
Comments